To achieve “the green shift” the world needs new materials, not least metals. Today, carbon is particularly popular in the metal industry because it has the ability to remove oxygen from the ore so that we can get the metal out. In that process, CO2 is formed, the greenhouse gas that we must avoid in the future. At this time, we have started on the task of finding other ways to extract metals, but what alternatives do we really have?
To ensure a future with the development of solar and wind energy, weather-proof roads and cities around the world, we must be able to make metals in a safe, climate-neutral and responsible way. Of course, we must improve the handling, and reusing or recycling of metal-containing waste, but that will not be enough. If we are to achieve the green shift, also globally, the world needs new metals. Today, the metal industry emits greenhouse gases and in Norway it accounts for 10 percent of the total CO2 emissions. It is therefore urgent to find new opportunities for more climate-friendly alternatives to current processes.
Three keys to future metal production
The figure (further down in the article) shows the substances that have the ability to remove oxygen from ores, these are called reducing agents. In the lower left corner of the figure, we see carbon that is used to convert almost all types of ore into metal, today. Historically, the carbon has been charcoal or wood, but in modern times it is mostly fossil carbon that is used. Organic substances such as wood and other forms of biocarbon are not pure forms of carbon, these are found on the line between carbon and hydrogen. Here we also find nontraditional biological carbon sources such as biogas.
Many metal producers see these carbon-based reducing agents as the most interesting reducing agents because they are expected to be able to adapt to current production methods, rather than developing completely new processes. However, the use of all carbon-containing reducing agents will lead to the formation of CO2. In order to prevent this from leading to an increase in the greenhouse effect, one must either use CO2-neutral carbon sources (e.g. biocarbon) or capture and store the CO2 from the exhaust gases. If we manage to do both at the same time, we can have CO2-negative processes in the future, which many believe will be necessary to achieve the climate targets. However, the so-called carbon neutral solutions also have a delay, as it takes an average of 90 years for a new tree to grow up and consume the CO2 emitted. This is too late if the goals of the Paris Agreement’ are to be reached.
A need for huge amounts of carbon
The big question regarding carbon is how the access to biocarbon will be like in the future. In addition, we must also think about the impacts on biodiversity and other important environmental aspects. The amounts of carbon needed within the metal sector are, unfortunately, huge. From a long-term perspective, carbon may not even be the best option we can find.
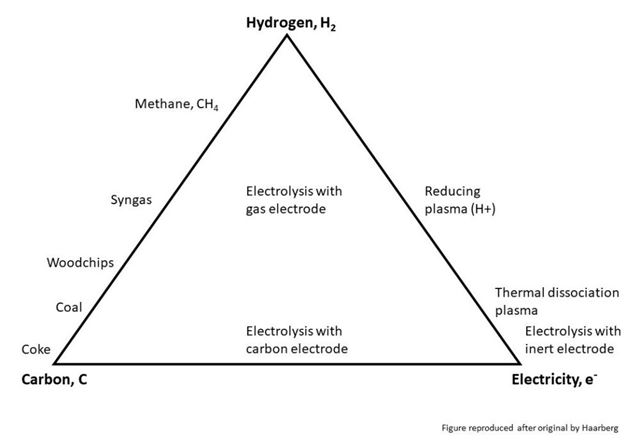
The figure shows a map of possibilities of which substances that have the ability to remove oxygen from ores, those called reducing agent.
Can energy replace reducing agents?
In the lower right corner of the figure, we have electricity. Many know that aluminium, for example, is made by electrolysis. Then electricity is used to get the oxygen in the ore to let go of the metal. However, today’s electrolysis technology lies somewhat towards the carbon corner of the figure, as electrodes made of carbon are needed in the electrolysis. The carbon electrodes are consumed in the process so that one actually combines the effects of electricity and carbon. If we can use other types of electrodes in the future, and then preferably types that are not used up in the process, the metal could, in theory, be made with only electricity as the reducing agents. Today, large resources around the world are spent on inventing new electrode solutions for different electrolysis processes for different metals. Energy optimists envisions a future with large amounts of renewable energy available, and if these forecasts turn out to be correct, such energy-intensive processes will become even more attractive from both an economic and environmental perspective.
If we take a closer look at the electron corner of the figure, we see that there is actually another possibility there: plasma. In fact, if unlimited amounts of energy could be used, it would be possible to make metal with no reduction agent other than clean energy. But then an extreme amount of energy is required, and this option would probably only be possible in the most optimistic energy scenarios.
Highly interesting hydrogen
In the upper corner of the figure, we find hydrogen. Hydrogen is particularly interesting for many reasons, especially since hydrogen is readily available as it is a by-product from several different industrial processes. Unfortunately, it is not possible to use all the hydrogen available today, primarily due to challenges related to transport, storage and safety. Hydrogen can also be made from natural gas, biogas or water (via electrolysis). Unfortunately, however, hydrogen does not have the ability to convert all types of ores into metal, but maybe there are ways to combine hydrogen with other reducing agents to make it more potent?
Hydrogen can cooperate
On the triangle’s right edge there is another high-energy alternative: hydrogen plasma. Here, so much energy is added that the hydrogen atoms have disintegrated. Hydrogen plasma is much more potent as a reducing agent than regular hydrogen gas and can be used on many more ores. This requires more energy than for gas reactions, but considerably less than if plasma is made from the ore itself. Another future possibility revolves around gas electrodes for electrolysis processes. Here it can be envisaged that hydrogen gas is used as a reducing agent in an electrolysis cell.
Another strong candidate for such gas electrodes is methane gas. A gas that is currently most easily obtained from natural gas, but which in the future may come from biological sources, i.e. biogas. Methane can either be a way to add hydrogen to a process, or it can be a reducing agent in itself. The combination of hydrogen and carbon in methane makes this a very interesting option for making metal in the future.
Other gases that can be used to add hydrogen to processes are ammonia, which, like methane, is less explosive than pure hydrogen and therefore easier to transport and store. But using gas as a reducing agent places great demands on restructuring the metal industries, where development and investment of new types of reactors would be necessary.
Can metals make metals?
We should also mention that many metals can be a reducing agent for other metals, although it is not included in the figure here. For example, aluminium can be a reducing agent for many other metals and for silicon. (Silicon is used in solar cells and electronics, for example). The problem with this though, is that you first have to produce aluminium, which currently does not happen without CO2 emissions. This category of metal production will therefore entirely depend on us putting in place methods both to make and recycle the new reducing agents in a climate-neutral way.
Why not recycle the carbon?
Speaking of recycling, recycling carbon is a kind of “holy grail”. It would be an extremely interesting option, as carbon can be produced without fossil raw materials. At the same time recycling would help reduce the pressure on biological carbon sources, such as forests. If we could capture CO2 from the exhaust gas and then split it up into oxygen gas, which can be released, and a carbon form that can be put back into furnaces and/or electrolysis cells, it would have been a very attractive solution.
The challenge is that CO2 is so incredibly stable that enormous amounts of energy will be needed to split it up. The amount of energy can possibly be slightly reduced by using high-tech catalysts, such as Cerium-containing particles or biological organisms such as algae or bacteria.
In other words, there are many different alternatives that all have both advantages and disadvantages. However, there is a good reason to believe that some of these alternatives could be the key to realizing a climate-neutral metal industry of the future.
Facts about the varied substances that can be used in metal production:
Hydrogen:
Hydrogen itself is carbon-free, but it can be produced in many different ways where not all are equally environmentally friendly. Therefore, there exists several different terms which tells something about how the hydrogen is produced.
Grey hydrogen: Hydrogen produced from natural gas, with an emission of 8 tons CO2 per ton hydrogen. Most of the hydrogen in use today is produced in this way.
Blue hydrogen: Hydrogen produced from natural gas with capture and storage of CO2. Some CO2 is emitted during the production of natural gas, and capture and storage is not 100 percent efficient.
Green hydrogen: Hydrogen which is produced close to 100 percent without CO2 emissions. The most common way is by using renewable electricity to split water into hydrogen and oxygen.
(Turquoise hydrogen: Hydrogen produced from natural gas where the carbon part is not converted to CO2, but instead preserved as a separate product, so-called "carbon black". This can be used in e.g. car tires.)
Plasma:
Plasma is, together with solids, liquids, and gas, one of four conditions in which a substance can occur in, a "fundamental state of matter".
In the same way as a liquid will boil and transform into gas when heated, a gas will transform again, into plasma form,when heated enough. Water boils at 100 °C, but water vapor does not switch to plasma form until closer to 12000 °C! However, one can lower the transition temperature with the help of electric power (this is what is done in fluorescent tubes. You send electricity through a gas and create plasma, and when the plasma returns to the gas form, it emits light). In plasma form, a substance splits into its smaller building blocks. For example, water (H2O) will be separated into hydrogen and oxygen components. The atoms in a plasma are very reactive and may react with substances where there would be no reaction in other states. For metal production, this is relevant in that a plasma can react with the oxygen in an ore and leave clean metal. One can also imagine making plasma from an ore, thus separating oxygen and the metal atoms, but this will require a lot of energy.
Biocarbon:
Most of the carbon used industrially today is of fossil origin, e.g. coal, oil and natural gas. All CO2 emitted from such carbon in an industrial process comes as an addition to the natural carbon cycle, and contributes to the increase of the CO2 concentration we have seen since pre-industrial times.
By biocarbon it is meant carbon of biological origin, e.g. from trees or agricultural waste. If there is not more biocarbon harvested than what naturally grows, such carbon will be part of the natural carbon cycle, and will not lead to a change in CO2 in the atmosphere over time. However, some people point out that it may take up to 90 years for a tree to grow to full size and that it therefore would then be wrong to look at biocarbon as a solution to carbon neutrality by 2050.
Some keywords:
- Biocarbon: Despite the fact that the name may seem to indicate all carbon of biological origin, biocarbon is usually used in the sense of "biochar", i.e. charcoal.
- Bio-oil: A liquid by-product from the production of charcoal. It contains many of the same components as fossil oil.
- Biogas: A gas with similar composition as natural gas (methane and carbon dioxide) that is formed by decomposition processes of biological waste.
Electrolysis:
Electrolysis is a chemical reaction in which elements can be separated by electric power. By sending power through an electrically conductive solution; electrolyte, the different elements will be deposited on separate electrodes, depending on the electrical voltage in use.
Basically, the purpose of the electrodes is only to supply electricity. But, sometimes the process is designed so that the electrode is intended to take part in the reaction. This is the case in aluminum production where carbon anodes not only supply electrical energy, but also supply chemical energy in a form of carbon that is converted into CO2 in the process. An electrode that does not participate in the process at all, a so-called inert anode, will eliminate these CO2 emissions by replacing the chemical energy from carbon with even more electrical energy. However, it is very challenging to construct completely inert anodes.
Since the electrolyte must be liquid, the metal production must either have very high temperatures (to melt the ore), or some strongly reactive solvents must be used (to dissolve the ore). A combination of these can also be used. Very few materials resist such conditions (high temperature, electrical voltage and/or reactive solution), and the electrodes that are used will slowly decompose.
Zinc, nickel and aluminium are examples of important metals that are currently produced via electrolysis.